A boundary layer is formed on the surface of a body due to friction, which causes the velocity of the surrounding flow to adapt to the velocity of the body surface. This boundary layer causes a considerably lower friction drag in the laminar (layer-like, calm) state than in the turbulent (turbulent, mixing) state. In aerodynamics, the laminar boundary layer in arrowed wings is already three-dimensional, and the laminar-to-turbulent transition takes place differently than in 2D boundary layers. At supersonic velocities, there is also a temperature boundary layer due to the large frictional heat. The instability mechanisms, the turbulent flow and influences/control methods, such as for instance roughness, wall temperature, pressure gradient, injection, suction, creation of controlled longitudinal vortices, plasma actuators, are investigated by Direct Numerical Simulations (DNS) with our own research code on supercomputers.
Research Projects
Control of 3-d boundary-layer transition
The basic mechanism in laminar-turbulent transition of 3-d boundary layers like on a swept wing or wind-turbine blades is crossflow instability. It leads to growing steady or travelling crossflow vortices that trigger turbulence by secondary instability. Control of instability and receptivity is investigated by application of spanwise rows of roughness, suction, or plasma actuators, the latter exerting a localized volume force on the flow.
Further reading:
VENKATA, S.Y., HEHNER, M.T., SERPIERI, J., BENARD, N., DÖRR, P.C., KLOKER, M.J., KOTSONIS, M. (2018) Experimental control of swept-wing transition through baseflow modification by plasma actuators. J. Fluid Mech. 844 R2-1-11, http://dx.doi.org/10.1017/jfm.2018.268.
DOERR, P.C., GUO, Z., PETER, J.M.F., KLOKER , M.J. (2018) Control of traveling crossflow vortices using plasma actuators. In: High Performance Computing in Science and Engineering ´17 (eds. W.E. Nagel, D.B. Kröner, M.M. Resch), Transactions (peer-reviewed) of the HLRS 2017, 289-306, Springer ; DOI 10.1007/978-3-319-68394-2_18.
DOERR, P.C., KLOKER, M.J. (2018) Numerical Investigations on Tollmien-Schlichting-Wave Attenuation Using Plasma Actuator Vortex Generators. AIAA Journal; 56: 1305-1309, 10.2514/1.J056779.
DOERR, P.C., KLOKER, M.J., HANIFI, A. (2017) Effect of Upstream Flow Deformation Using Plasma Actuators on Crossflow Transition Induced by Unsteady Vortical Free-Stream Disturbances. AIAA-2017-3114.
DOERR, P.C., KLOKER, M.J. (2017) Crossflow transition control by upstream flow deformation using plasma actuators. J. Appl. Phys. 121, 063303 (2017), http://dx.doi.org/10.1063/1.4975791.
DOERR, P.C., KLOKER, M.J. (2016) Transition control in a three-dimensional boundary layer by direct attenuation of nonlinear crossflow vortices using plasma actuators. Int. J. Heat Fluid Flow 61 (B), 449-465; 10.1016/j.ijheatfluidflow.2016.06.005.
DOERR, P.C., KLOKER, M.J. (2015) Numerical investigation of plasma actuator force-term estimations from flow experiments. J. Phys D: Appl. Phys. 48 (2015) 395203.
DOERR, P.C., KLOKER, M.J. (2015) Stabilisation of a three-dimensional boundary layer by base-flow manipulation using plasma actuators. J. Phys D: Appl. Phys. 48 (2015) 285205.
FRIEDERICH, T., KLOKER, M.J. (2012) Control of the secondary crossflow instability using localized suction. J. Fluid Mech. 706, 470-495.
MESSING, R., KLOKER, M.J. (2010) Investigation of suction for laminar flow control of three-dimensional boundary layers. J. Fluid Mech. 658, 117-147.
WASSERMANN, P., KLOKER, M.J. (2002) Mechanisms and passive control of crossflow-vortex-induced transition in a three-dimensional boundary layer. J. Fluid Mech. 456, 49-84.
Receptivity of the boundary layer on a swept wing at low and high Re number
The fundamental receptivity to wall roughness and external disturbances is investigated in the boundary layer on a swept wing subject to crossflow instability. The knowledge of the maximum allowable roughness to not cause sudden transition to turbulence is a prerequisite for laminar flow control on a swept wing. Oncoming external disturbances are squeezed near the attachment line and the process which parts are taken up by the crossflow boundary layer is complex. The DNS strive to clarify these points at low and high Re number, including compressibility effects.
Further reading:
DOERR, P.C., KLOKER, M.J., HANIFI, A. (2017) Effect of Upstream Flow Deformation Using Plasma Actuators on Crossflow Transition Induced by Unsteady Vortical Free-Stream Disturbances. AIAA-2017-3114.
KURZ, H.B.E., KLOKER, M.J. (2016) Mechanisms of flow tripping by discrete roughness elements in a swept-wing boundary layer. J. Fluid Mech. 796, 158-194.
KURZ, H.B.E., KLOKER, M.J. (2016) Receptivity of a swept-wing boundary layer to steady vortical free-stream disturbances. In: New Results in Numerical and
Experimental Fluid Dynamics X (eds. A. Dillmann et al.), NNFM 132, peer-reviewed contributions to the 19. STAB/DGLR-Symposium, Munich, Nov. 2014, 227-236, Springer.
KURZ, H.B.E., KLOKER, M.J. (2015) Swept-wing boundary-layer receptivity to steady free-stream disturbances. AIAA-2015-3079.
KURZ, H.B.E., KLOKER, M.J. (2015) Discrete-roughness effects in a 3-d boundary layer on an airfoil investigated by DNS. Procedia IUTAM 14, IUTAM ABCM Symposium on Laminar Turbulent Transition, Rio de Janeiro, Brazil, Sept. 2014, 163-172, Elsevier.
KURZ, H.B.E., KLOKER, M.J. (2014) Receptivity of a swept-wing boundary layer to micron-sized discrete roughness elements. J. Fluid Mech. 755, 62-82.
Film cooling in supersonic boundary layers
Modern rocket nozzle extensions have to be actively cooled in addition to cooling channels below the wall. A cooling gas is injected normally or tangentially into the supersonic flow, in the latter case also with supersonic velocity. The resulting shear-flow field and the wall heat-load protection can be very complex depending on many parameters like the axial pressure gradient, the hot, main-gas boundary-layer state – laminar or turbulent –, the injection slot height, the step height, the cooling gas type, over- or underexpanded cooling jet.
Further reading:
WENZEL, C., SELENT, B., KLOKER, M.J., RIST, U. (2018)
DNS of compressible turbulent boundary layers and assessment of data-/scaling-law quality. J. Fluid Mech. 842, 428-468, http://dx.doi.org/10.1017/jfm.2018.179.
PETER, J.M.F., KLOKER, M.J. (2017) Preliminary work for DNS of rocket-nozzle film cooling. DLRK-2017-450178, urn:nbn:de:101:1-201710202849
KELLER, M., KLOKER, M.J. (2016) Direct numerical simulation of foreign-gas film cooling in supersonic boundary-layer flow. AIAA Journal 55, no. 1, 99-111; http://arc.aiaa.org/doi/abs/10.2514/1.J055115.
KELLER, M., KLOKER, M.J., OLIVIER, H. (2015) Influence of cooling-gas properties on film-cooling effectiveness in supersonic flow. J. Spacecraft and Rockets 52, no. 5, 1443-1455; http://arc.aiaa.org/doi/abs/10.2514/1.A33203.
KELLER, M., KLOKER, M.J. (2014/15) Effusion cooling and flow tripping in a laminar supersonic boundary-layer flow. AIAA Journal 53, no. 4, 902-919; http://arc.aiaa.org/doi/abs/10.2514/1.J053251.
KELLER, M., KLOKER, M.J., KIRILOVSKIY, S., POLIVANOV, P., SIDORENKO, A., MASLOV, A. (2014) Study of Flow Control by Localized Volume Heating in Hypersonic Boundary Layers. CEAS Space Journal 6, Issue 3, p. 119-132.
KELLER, M.; KLOKER, M.J. (2013) Direct Numerical Simulations of Film Cooling in a Supersonic Boundary-Layer Flow on Massively-Parallel Supercomputers. In: Sustained Simulation Performance 2013 (eds. M.M. Resch, W. Bez, E. Focht, H. Kobayashi, Y. Kovalenko), 107-128, Springer.
KELLER, M., KLOKER, M.J. (2011) Influence of a favorable streamwise pressure gradient on laminar film cooling at Mach 2.67, Proc. 4th European Conference for Aerospace Sciences (EUCASS 2011), 10 pages, http://eucass.ru/cs/index.php/eu/2011, see ResearchGate.
Transition in hypersonic flow
In hypersonic flow, with the Mach number larger than 4, wall heating is a critical issue. The flow tends to be longer laminar in terms of the transition Reynolds number. However, surface roughness and a special disturbance mode, caused by a relative supersonic region near the wall where the disturbances travel faster than the fast sound wave, can lead to early turbulence. Understanding, avoiding or controlling hypersonic transition enables not to overdesign the thermal protection necessary due to safety reasons, and the payload ratio can then be larger.
Further reading:
GROSKOPF, G., KLOKER, M.J. (2016) Instability and transition mechanisms induced by skewed roughness elements in a high-speed boundary-layer. J. Fluid Mech. 805, 262-302, http://dx.doi.org/10.1017/jfm.2016.563.
GROSKOPF, G., KLOKER, M.J. (2012) Stability analysis of three-dimensional hypersonic boundary-layer flows with discrete surface roughness, NATO-RTO-MP-AVT-200-30:1-19.
GROSKOPF, G., KLOKER, M.J., STEPHANI, K.A. (2011)Temperature / rarefaction effects in hypersonic boundary-layer flow with an oblique roughness element, AIAA-2011-3251.
LINN, J., KLOKER, M.J. (2010) Investigation of thermal nonequilibrium on hypersonic boundary-layer transition by DNS. In: Laminar-Turbulent Transition (eds. P. Schlatter, D. Henningson), 7th IUTAM-Symposium, Stockholm, Sweden (2009), 521-524, Springer.
FEZER, A., KLOKER, M.J. (2003) DNS of transition mechanisms at Mach 6.8 – flat plate vs. sharp cone. In: West East High Speed Flow Fields 2002 (eds. D.E. Zeitoun, J. Periaux, J.A. Desideri, M. Marini), Proc. W.E.H.S.F.F. conference, Marseille, France, April 22-26, 2002, 434-441,CIMNE (Barcelona, Spain).
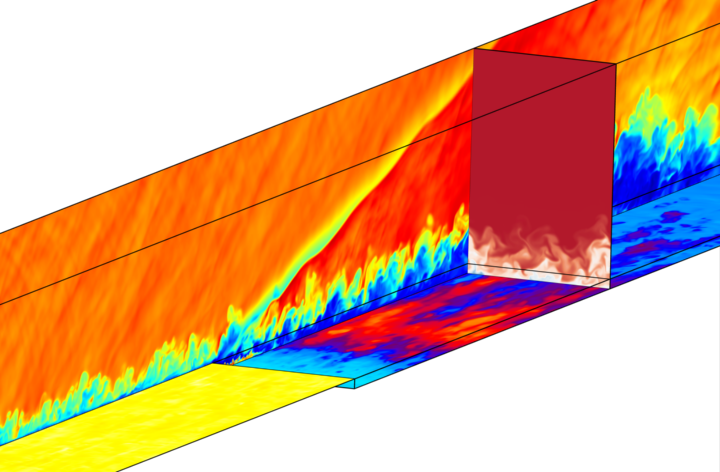
Dr.-Ing. Markus J. Kloker
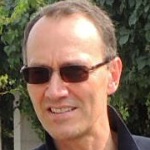
Markus J. Kloker
Dr.-Ing.Head of working group Transition and Turbulence